The origin of mineral color
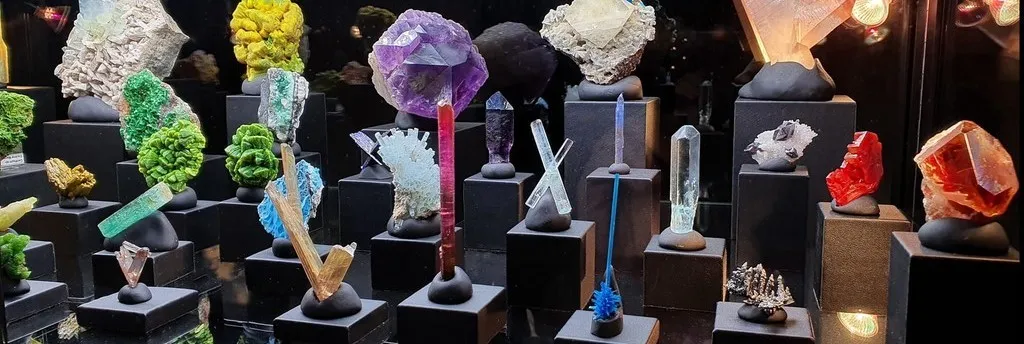
Minerals, with their fascinating and varied colors, have always captured the attention of curious people and scientists. From the deep green of emerald to the deep blue of azurite, these vibrant shades are the result of a subtle marriage of physics, chemistry, and crystal structure. But what explains these unique colors? Let's explore the secrets of mineral coloring together.
Light and minerals : a colorful encounter
Selective absorption of light :
The color of minerals comes from their interaction with visible light, the part of the electromagnetic spectrum that our eyes can perceive (between 350 and 750 nanometers). When a ray of light hits a mineral, part of this light is absorbed, and the other part is reflected or transmitted. What we see corresponds to the unabsorbed wavelengths, that is, those that are re-emitted by the mineral.
Consider the example of chlorophyll in plants. It absorbs red and blue light for photosynthesis, but reflects green light, thus explaining the color of the foliage. Similarly, each mineral has a unique light absorption signature, dictated by its chemical composition and crystal structure.
Characteristics of light :
Light is an electromagnetic wave characterized by its wavelength. The colors of the visible spectrum range from red (long wavelength, low energy) to violet (short wavelength, high energy). When it interacts with electrons in minerals, it can cause energy transitions, absorbing some wavelengths and leaving others visible.

The origins of color
Transition elements :
Minerals often get their colors from transition elements, metals such as iron (Fe), copper (Cu) or chromium (Cr). These elements have electrons in d atomic orbitals (or f for actinides and lanthanides), which can be excited by light. These excitations, called d-d transitions, absorb specific wavelengths, thus giving a characteristic color to the mineral. For example:
Color centers :
Color centers are anomalies in the crystal structure of minerals that influence their color. These defects, often called F centers (from the German farbe, color), occur in a variety of ways :
- Ionic vacancies : When an ion is missing from the crystal structure, an electron can occupy the vacancy. For example, in fluorite, fluorine ion vacancies trap electrons that absorb specific wavelengths, producing a violet hue.
- Ionic substitutions : When an ion replaces another in the structure but with a different oxidation state, this can create color defects. In amethyst, Fe3+ ions substitute for silicon, causing anomalies that absorb certain wavelengths and create a violet coloration.
- Interstitial impurities : Foreign elements inserted between atoms can also interact with light and generate unique colors.
Charge transfers :
Charge transfers are another fundamental mechanism in the coloration of minerals. This phenomenon occurs when an electron is transferred between two neighboring ions or atomic groups, thus changing the way the mineral interacts with light.
Ion-ion transfers :
An ion-ion charge transfer generally involves two ions of different valences, such as Fe2+ and Fe3+, or Fe2+ and Ti4+. When an electron is exchanged between these ions under the effect of light, certain wavelengths are absorbed. This phenomenon is what gives sapphire its intense blue hue, resulting from exchanges between iron and titanium.
Group-ion transfers :
In some minerals, such as vanadinite or crocoite, the charge transfer occurs between a group of atoms (for example, V5+-O4 or Cr6+-O4). These transfers absorb specific wavelengths, creating vibrant hues ranging from yellow to red. For example:
- Vanadinite : Absorption in the blue produces a yellow-orange hue.
- Crocoite : Transfer between Cr6+ and O- produces an intense red.
Internal-external transfers :
Some minerals exhibit electron transfer between an atom in the crystal and a foreign inclusion or structural defect. This type of transfer is often accompanied by fluorescence, as in the case of uraninite, which emits a yellow-green glow.
In short, charge transfers add a dynamic dimension to the coloration of minerals, directly linking their chemical composition to their unique visual appearance.
Optical phenomena : the magic of interactions
Diffraction :
Diffraction occurs when light is deflected as it passes through or around periodic structures in a mineral. This phenomenon is the origin of the play of colors in opals. This mineral is composed of microscopic spheres of silica arranged in a regular pattern. When a ray of light interacts with these spheres, the different wavelengths of light are scattered at different angles, creating a rainbow of colors. The larger the spheres, the more the colors tend toward red; the smaller they are, the more the hues turn toward blue.
In some cases, such as labradorite, the iridescence results from the microscopic superposition of alternating layers of calcium and sodium feldspar (perthites). Diffraction through these layers produces flashes of color that change depending on the angle of observation. Iridescence on metallic minerals is also linked to this phenomenon by the presence of a very thin film of transparent oxides on their surfaces (iridescent goethites for example).
Refraction :
Refraction is the change in direction that a light wave undergoes when it passes through a medium with a different refractive index. This phenomenon is responsible for the decomposition of white light by prisms or in rainbows. When it passes through transparent minerals such as diamond or quartz, refraction can create dispersion effects that separate the constituent colors of the light. Cut diamonds, for example, exploit this phenomenon to produce multi-colored lights, amplifying their natural beauty.
Diffusion :
Diffusion occurs when light is scattered in all directions by small particles or inclusions in a mineral. This phenomenon is at the origin of the blue color of lussatite and certain milky quartz. In the case of the sky, Rayleigh scattering explains why blue, having a short wavelength, is more dispersed than other colors. For minerals, the size and nature of the included particles directly influence the perceived color. For example :
- Rose quartz : Microscopic inclusions of dumortierite scatter light, creating a soft and elegant pink hue.
- Blue quartz : Increased scattering of red and green wavelengths lets blue tones predominate.
When the particles are larger than the wavelength of visible light, all colors are scattered equally, giving a white or grayish appearance, as in snow or salt.
Elastic optical phenomena, such as diffraction, refraction and diffusion, enrich the color palette of minerals without altering their chemical composition. They fully participate in the visual magic of natural stones, transforming the play of light into wonder.
Conclusion
The color of minerals, the result of a subtle balance between chemistry, physics, and crystal structure, represents much more than just aesthetics. It reflects complex interactions between light and matter : electrons jump between energy levels, crystal defects modify light absorption, and optical phenomena transform light rays into dazzling spectacles.
Beyond their beauty, colored minerals remind us of the wonders of nature and the fundamental laws that govern our universe. Understanding these mechanisms only amplifies our admiration for these flashes of light frozen in rock, witnesses to the history of the Earth.
References :
Emmanuel Fritsch : « La couleur des minéraux et des gemmes », Monde et minéraux N°67, 39 et 70 (1985).
Steven Dutch : « Why Minerals Are Colored », Natural and Applied Sciences, Université de Wisconsin-Green Bay.
Cours de l'Institut de Gemmologie Américain (GIA)