The Colombian trapiche emeralds
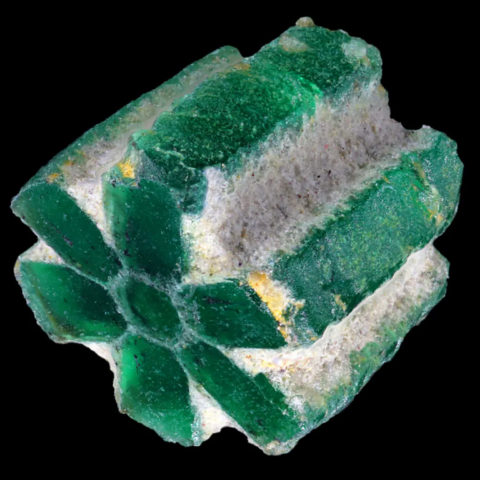
Among the mineralogical curiosities associated with Colombian emerald, trapiche emerald is undoubtedly the most emblematic. This rare stone, recognizable by its singular texture, is much admired by gem enthusiasts, as it is by scientists. Its formation is linked to physico-chemical variations during its crystalline growth, making trapiche emerald an important geological marker of deposits located in the western emerald belt of Colombia's Cordillera Oriental.
The term “trapiche” was introduced to describe emerald samples with a texture reminiscent of the cogwheels used to crush sugarcane. These wheels, traditionally used in mills to extract the juice from the cane, have a distinctive serrated structure, evoking the radial sectors visible in trapiche emerald crystals. This analogy illustrates not only the visual appearance, but also the functional and ingenious nature of natural systems imitating mechanical forms. In section perpendicular to the crystallographic axis, these crystals reveal a hexagonal core surrounded by six growth sectors separated by inclusion-rich zones. These aesthetic and geological features make them a true wonder of nature.
Photo : Trapiche emerald crystal from Muzo, Colombia © Martin Slama
Geological context
Trapiche emeralds are formed in an exceptional geological setting, within the Cordillera Oriental basin in Colombia. This region, situated at the crossroads of major tectonic structures, is marked by thick, folded and faulted Mesozoic sedimentary series. These formations are the result of tectonic inversion of the subsiding marine basin in the Middle Miocene, around 15 million years ago, during the Andean tectonic phase. Tectonic inversion refers to a geodynamic process whereby a region that was initially in subsidence - i.e. a marine basin that was subsiding under the weight of accumulated sediments - undergoes tectonic compression. This compression causes the uplift and folding of sedimentary strata, transforming an ancient basin into a mountain range. In the case of the Cordillera Oriental, this inversion led to the surrection of the mountain range, separating the Llanos Foreland basin to the east and the Middle Magdalena basin to the west, and created an ideal environment for the circulation of hydrothermal fluids.
Simplified geological map : Eastern Cordillera Basin in Colombia from Pignatelli & al. (2015)
Emerald deposits are divided into two main belts. The eastern belt includes the mining districts of Chivor, Gachalá and Macanal, where mineralization is contained in the Berriasian limestones and black shales (-135 to -130 MY) of the Guavio Formation, overlain by the siliceous black shales of the Macanal Formation (-130 to -122 MY). By contrast, the western belt, home to the famous Muzo, Coscuez, Peñas Blancas and La Pita deposits, is associated with Valanginian-Hauterivian formations (-130 to -116 MY) consisting of dolomitic limestones and carbon-rich black shales of the Rosablanca and Paja Formations.
In both belts, emerald mineralization is closely linked to hydrothermal activity, facilitated by the presence of black shales rich in organic matter and sulfates of evaporitic origin. These geological conditions have enabled hot brines to interact with the surrounding rocks. These fluids, characterized by a complex composition, are saturated with salt (NaCl) (~40% by weight) and formed at temperatures of 300-330°C. The reduction of sulfates by organic matter in black shales generated hydrogen sulfide (H2S), responsible for the precipitation of pyrite, and bicarbonate (HCO3-), involved in the formation of carbonates such as calcite and dolomite. This reductive chemistry, combined with the enrichment of trace elements such as chromium and vanadium, favoured emerald crystallization.
The formation of trapiche emeralds is directly influenced by the structural and tectonic context. Phases of tectonic compression, followed by hydraulic fracturing due to fluid overpressure, led to the creation of extensional vein networks and stratiform breccias, where emeralds precipitated. These processes took place over distinct periods depending on the belt : at the Cretaceous-Tertiary boundary (around -65 MY) in the eastern belt and at the Eocene-Oligocene transition (-38 to -32 MY) in the western belt, as shown by the dating of associated green muscovite. These events testify to the complex tectonic-sedimentary evolution of the region and explain the location and unique characteristics of the Colombian deposits.
The geological features of Colombian emeralds, such as halite-rich multiphase fluid inclusions, reflect the dynamics of these hydrothermal environments. The combination of tectonic, sedimentary and chemical conditions not only gave rise to these exceptional gems, but also enabled the formation of their unique variants, such as trapiche emeralds, emblematic of the region's mineralogical wealth.
Research history
Trapiche emeralds have attracted the interest of researchers since the end of the 19th century, when Émile Bertrand first mentioned them in 1879, describing their unique texture without understanding how they formed. This initial observation was followed by the work of Bergt (1899) and Codazzi (1915), who put forward various hypotheses, including a resemblance to the cyclic twinning of aragonite. However, Pogue (1916) proposed an explanation that focused more on the specific crystallization conditions that gave rise to this unique structure.
In the 1930's, Bernauer made a significant breakthrough by studying samples from the famous Muzo mine, where he identified various inclusions such as quartz, muscovite, albite, pyrite, as well as organic matter responsible for the black coloration in the crystals. His observations laid the foundation for subsequent studies, which revealed multiphase fluid inclusions and secondary minerals, providing valuable information on the hydrothermal processes of emerald formation. These discoveries were linked to major tectonic events that shaped the region, reinforcing the understanding of the specific geological contexts of the Colombian Emerald Belt. In 1964, McKague proposed a genetic model that integrated variations in pressure, temperature and chemical composition of the mineralizing solutions.
Modern research has confirmed that trapiche emeralds are exclusively associated with the mines of the western Emerald Belt, particularly those of Muzo and Peñas Blancas. No specimens have been discovered in the districts of Chivor and Gachalá, highlighting the geographical and geological uniqueness of these fascinating stones.
The structure of a trapiche emerald crystal
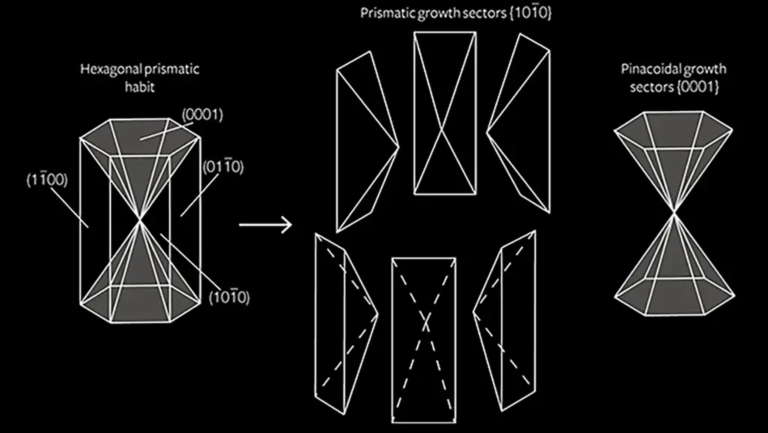
Habit of emerald crystals, characterized by six prismatic faces {1-100} and two pinacoidal faces {0001} and their growth sectors according to Rakovan & al. (2006)
The structure of a trapiche emerald crystal

Traphiche emeralds exhibit a unique crystal architecture, characterized by distinct elements that are arranged in a hexagonal pattern. The main parts of a trapiche emerald crystal include the core, arms, dendrites, and overgrowth zones.
The central core :
The core is the central part of the crystal and may be present or absent in a trapiche emerald. It is delimited by pinacoidal faces {0001} and has a hexagonal bipyramidal shape, corresponding to the pinacoidal growth sectors. The size and shape of the core vary according to the cross section; in sections perpendicular to the c-axis, the size of the core depends on the position on the hexagonal double pyramid. Near their bases, it appears larger, while it becomes smaller or disappears near the apex. When a section is taken through the crystal between the vertices of the two opposing pyramids of the pinacoid, the core is absent, and the arms and dendrites intersect at a central point. The core may also undergo dissolution processes during its formation, changing its shape from hexagonal to oval, and exhibiting irregular borders. These dissolution events are often accompanied by fracturing and plastic deformation, visible under cross-polarized light. The color of the core varies from pale to dark green, depending on the concentration of chromophores such as Cr3+ and V3+.
Arms :
Six hexagonal arms surround the core, arranged symmetrically along the {110} crystallographic directions. They are defined by the {1-100} prismatic faces and often appear elongated in these directions. Although the arms are generally of similar size, asymmetries may occur, with some arms being more developed. Their color varies from pale green to intense green, depending on the chemical composition, especially the trace elements present. Each arm contains bundles of straight dislocations, perpendicular to the {1-100} faces. These dislocations, formed during crystal growth, trap solid and fluid inclusions, often aligned parallel to the dislocations. The multiphase inclusions, rich in halite, carbonates, and sylvite, reflect the hydrothermal conditions during crystallization. The arms may have fractures filled with albite and small beryl crystals, without disturbing their growth. Some arms have a fibrous texture, similar to that of dendrites, indicating a rapid growth rate.
Dendrites :
Dendrites surround the nucleus and extend radially outward. They have a herringbone texture near the nucleus, which evolves into a characteristic fan shape towards the edges of the crystal. This texture can vary from one sample to another, with dendrites wider or containing crossed beryl filaments at 60°. Dendrites are often composed of calcite, albite, and sometimes beryl. They are developed on a matrix of black albitized and calcitized schists. The presence of organic matter in the dendrites and matrix often gives them a dark color. Dendrites interact directly with the arms, sometimes penetrating them. They can also contain multiphase inclusions similar to those of the arms, which indicates a common hydrothermal fluid during crystallization.
Overgrowth zones :
Overgrowth zones form at the periphery of the crystal, marking the final stages of its development. They surround the dendrites and arms, completing the hexagonal structure. These zones are composed mainly of beryl, often transparent, and reveal a more uniform growth compared to the internal parts of the trapiche.
Formation of trapiche emerald crystals
Experimental investigations confirm that trapiche emerald is a single crystal and not a twin, as demonstrated by Pignatelli & al. (2015). X-ray topography images reveal a crystal continuity between the core and the prismatic growth sectors, eliminating the hypothesis of the coexistence of several crystals.
The formation of trapiche emeralds can be decomposed into three main stages, modified after Pignatelli & al. (2015) :
Stage 1 - Compressive tectonic context and initial formation
The formation of the emerald deposits of the Western Belt is associated with a compressional tectonic system (Branquet et al., 1999). This deformation causes the formation of folds and thrusts, accompanied by faults. The circulation of hydrothermal fluids in these structures generates a local increase in fluid pressure. These conditions create tension zones in the black schists, promoting fluid saturation and initial emerald precipitation. The core of the trapiche emeralds then forms, forming a base for the growth sectors.
Stage 2 - Hydraulic fracturing and acquisition of the trapiche texture
When fluid pressure exceeds lithostatic pressure, hydraulic brecciation occurs, causing a sudden decompression of the fluid overpressure zone. This decompression leads to rapid growth of prismatic sectors, forming the trapiche texture. The boundaries between these sectors develop from the core between the arms. These boundaries exhibit high growth rates, which explains the formation of the six characteristic dendrites. The growth of the prismatic sectors pushes the surrounding matrix except near the boundaries, where inclusions are trapped, creating the dark zones.
Stage 3 - Fluid System Stabilization
After tectonic decompression and hydraulic fracturing, the degree of fluid saturation decreases, allowing the fluid system to return to equilibrium. It is at this stage that overgrowth can form on the periphery of the trapiche crystal.
During these stages, the chemical composition of the fluids evolves, modifying the levels of trace elements such as chromium and vanadium. These chemical variations explain the compositional zonations observed in trapiche emeralds.
Conclusion
The trapiche emerald, with its unique texture and complex geological history, is much more than a mineralogical curiosity. It is a natural archive that documents the interactions between tectonics, hydraulic fracturing and hydrothermal processes. Its formation, closely linked to specific geodynamic conditions in the western emerald belt of Colombia, illustrates the crucial role of compressive tectonic systems and physicochemical variations of fluids.
Extensive research on trapiche texture and fluid inclusions has allowed us to reconstruct the pressure and temperature environments at the origin of these gems, while providing insight into the formation mechanisms related to hydraulic fracturing. These discoveries not only enrich our understanding of Colombian deposits, but also highlight the importance of these stones as geological markers.
At the crossroads of aesthetics and science, the trapiche emerald remains an inexhaustible source of wonder and investigation. It embodies an exceptional testimony to the forces that shape the Earth, while continuing to captivate gemologists, geologists and collectors around the world.
References :
BERGT W. (1899) Die älteren Massengesteine, kristallinen Schiefer und Sedimente, p. 46. In F. BERNAUER Ed., Compilación de los Estudios Geológicos Oficiales en Colombia -1917 a 1933, No. 4, pp. 199–221.
BERNAUER F., Ed. (1933) Las llamadas maclas múltiples de esmeralda de Muzo y sus anomalías ópticas. Compilación de los Estudios Geológicos Oficiales en Colombia -1917 a 1933, No. 4, pp. 199–221.
BERTRAND E. (1879) Compte-rendu de la séance du 13 Février 1879 [Report of the meeting of February 13, 1879]. Bulletin de la Société Minéralogique de France, Vol. 2, p. 31.
BRANQUET Y. (1999) Étude structurale et métallogénique des gisements d’émeraude de Colombie : contribution à l’histoire tectono-sédimentaire de la cordillère orientale de Colombie. PhD Thesis, Université de Lorraine, Nancy, France.
BRANQUET Y., LAUMONIER B., CHEILLETZ A., GIULIANI G. (1999a) Emeralds in the eastern Cordillera of Colombia: two tectonic settings for one mineralisation. Geology, Vol. 27, No. 7, pp. 597–600.
BRANQUET Y., CHEILLETZ A., GIULIANI G., LAUMONIER B., BLANCO O. (1999b) Fluidized hydrothermal breccia in dilatant faults during thrusting: The Colombian emerald deposits. In Fractures, Fluid and Mineralization. Geological Society, London, 1999, Special Publication 155, pp. 183–195.
BRANQUET Y., GIULIANI G., CHEILLETZ A., LAUMONIER B. (2015) Colombian emeralds and evaporites: tectono-stratigraphic significance of a regional emerald-bearing evaporitic breccia level. 13th SGA Biennial Meeting, Nancy, France, Proceedings Vol. 4, pp. 1291–1294.
CODAZZI R.L. (1915) Los minerales de Muzo. Contribución al Estudio de los Minerales de Colombia, Bogotá, pp. 3–7.
GIULIANI G. (2022). Émeraudes, tout un monde ! Éditions du Piat.
MCKAGUE H.L. (1964) Trapiche emeralds from Colombia. Gems & Gemology, Vol. 11, No. 7, pp. 210–223.
PIGNATELLI I., LAURS B. M., GIULIANI G., FALLICK A. E., & BARRA F. (2015) Colombian Trapiche Emeralds: Recent Advances in Understanding Their Formation. Gems & Gemology, 51(3), 222–245.
POGUE J.E. (1916) The emerald deposits of Muzo, Colombia. Transactions of the American Institute of Mining Engineers, Vol. 55, pp. 383–406.
RAKOVAN J., KITAMURA M., TUMADA O. (2006). Sakura ishi (cherry blossom stones): Mica pseudomorphs of complex cordierite-indialite intergrowths from Kameoka, Kyoto Prefecture, Japan. Rocks and Minerals, Vol. 81, No. 4, pp. 284–292
SUNAGAWA I. (1999) Growth and morphology of crystals. Forma, Vol. 14, pp. 147–166.